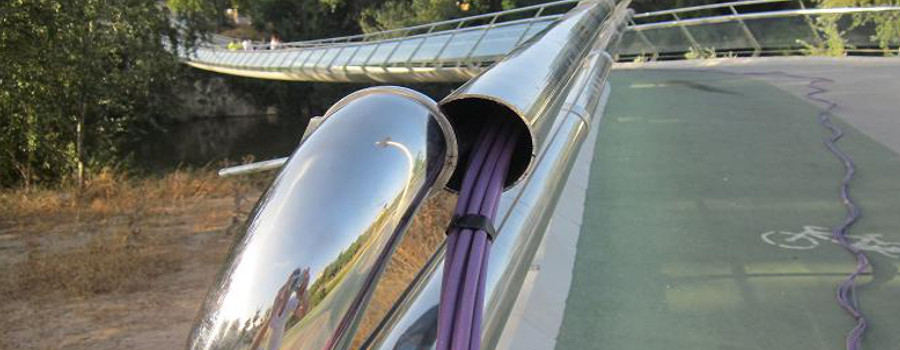
When structures age
How to reduce structural conservation task expenses by implementing monitoring systems?
The structures are not everlasting. They are projected to play a role for a certain number of years. Thus, a wind turbine mast lifespan is about 20 years while in the case of a bridge it depends on the type and the material used. According to Guy Grattesat, metal bridge will have a lifespan of 40 years, 100 years for a reinforced concrete, between 15 and 20 years for those made of wood and about 200 years for masonry. Nevertheless, exceeding the life expectancy does not necessarily mean dismantling the structure. Generally, what we do is a more comprehensive monitoring effort and implementing conservation works if necessary.
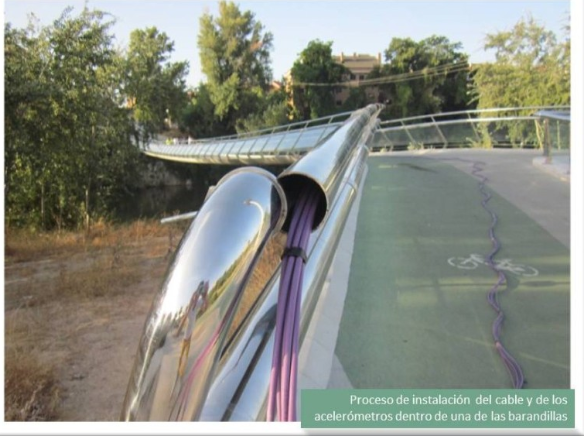
The European bridge stock is catching its lifespan and it is showing signs of fatigue. According to Eva Lantsoght, Professor of engineering at the University of San Francisco de Quito, “European bridges are old, but their replacement involves a great investment. Only in the Netherlands, there are about 3,000 bridges that could cause problems, being their refurbishment cost around one million euros each”. To mention another example, the first wind turbine parks (1984), whose technological emergence came in 2002 (according to the GWEC, Global Wind Statistics), have great needs for maintenance and the increase of these needs will have the same exponential curve, I can imagine, that its development has had in the past.
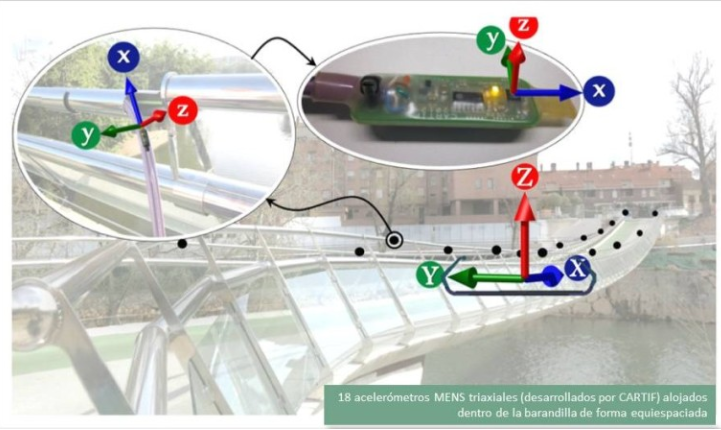
Having this background in mind, I would like to highlight the importance of implementing new maintenance strategies to reduce structural conservation task expenses. State-of-the-art monitoring systems, developed in recent years at increasingly low costs can be the solution. Usually in this type of structures, the procedure consists on the installation of a network of accelerometers to record the ambient vibration response of the structure. Using identification techniques, the modal characteristics can be estimated and be used to evaluate the structural integrity.
The idea is to know the structural behavior in operation conditions and to determine the valid range by controlling only a few parameters (frequency, mode and damping), being these parameters easy to evaluate and to record their trends along the time. Although the meaning of the word ‘monitoring’ refers to the capacity of the acquisition system to record certain values, it is also important to add the ability to process the data and to generate alarm codes, if it would be necessary.
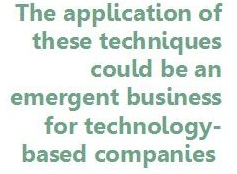
In this aim, researchers require a deep understanding of the technical matter involved as well as a big experience in experimental techniques and data processing. OMA (Operational Modal Analysis) identification techniques are based just on the acceleration records but if some loading signal applied on the structure could be also record, additional information can be obtained using EMA (Experimental Modal Analysis “EMA”). Both approaches should gradually replace, or at least complement, the traditional visual inspections and static loading tests. Monitoring systems could be an emergent business for technology-based companies in cooperation with new maintenance standards or strategies by the infrastructure’s authorities.
In this regard, I can mention the success of low-cost monitoring system of Pedro Gómez Bosque footbridge (built in Valladolid) operated by CARTIF team since 2012. This stress-ribbon structure undergoes no linear effects. The collected data has been useful so far to understand the behavior under dense pedestrian loading, at different met conditions (summer or winter, strong wind…), etc. Analyzing this information, we know what it is normal and what is not, so we can establish an optimal operational range and determine when anomalies appear and why. In these terms, the structure is an ideal benchmark not only for structural monitoring but also for understanding mechanical problems, pedestrian loading and other engineering issues.